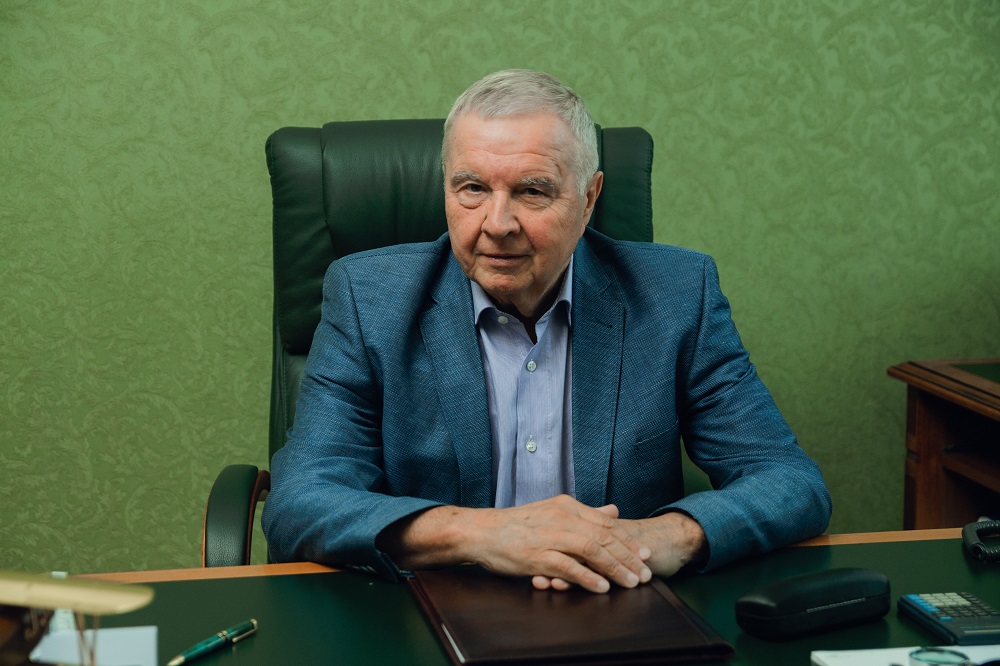
– Mr. Soifer, what was the prerequisite for this research into diffractive optics and nanophotonics? What are diffractive optics and diffractive lenses, and how do they differ from ordinary optical lenses used in glasses, binoculars, telescopes and cameras?
– Let me first give some clarification regarding the terminology. Everyone is familiar with the classic optical device – the lens. The British Museum houses a lens from Assyria that is more than 3,000 years old. The human eye has its own lens. An optical lens is a transparent body held together by two curved surfaces, for example, spherical surfaces. Various materials can be used to make lenses. Usually they are made from plastic or glass. A spherical lens refracts rays of light within its body, concentrating them at a point found at a certain distance behind it into a bright spot. This point is called the focus. The classic lens is often referred to as a refractive lens. The Aristophanes’ play "Clouds" (424 BC) describes how fire can be produced from sunlight using a convex glass.
Depending on the purpose, the thickness of a refractive lens can be quite significant, ranging from a few millimeters to dozens of centimeters. Large lenses can weigh several kilograms. Such bulky lenses are unsuitable when solving a number of practical tasks. In particular, they cannot be utilized onboard small space vehicles and drones. I’ll remind you that launching 1 kg of weight into space costs about 20,000 euros. Another common refractive device is a prism, which decomposes white light into a spectrum according to the different constituent wavelengths.
– Here we come to a fundamental question: is it possible to make a lens or prism flat and lightweight?
– It turns out that it is possible, if you remember that in addition to refraction, there is a phenomenon of diffraction. Diffraction (break, fracture – lat.) is when light bends around various obstacles, i.e. deviates from the laws of rectilinear ray propagation adopted in the geometric optics. The wave nature of light is evidenced in the phenomenon of diffraction. The phenomenon of diffraction was first described in 1673 by the monk James Gregory. He observed the dispersion of white light into a spectrum on a bird’s feather – a naturally occurring diffraction grating. In 1821, Fraunhofer created a diffraction grating from a thin wire and used it to study the spectra. In 1818, Fresnel developed the theory of light diffraction and actually proposed the idea of a flat lens, called a zone plate. A zone plate is composed of a large number of alternating black and white concentric rings of various widths which, at a certain wavelength, acts as a lens. For instance, if we aim to get a 4-fold increase in the light transmission intensity, the black rings need to be engraved to a depth of half the incident wavelength. This kind of zone plate is called an optical retarder.
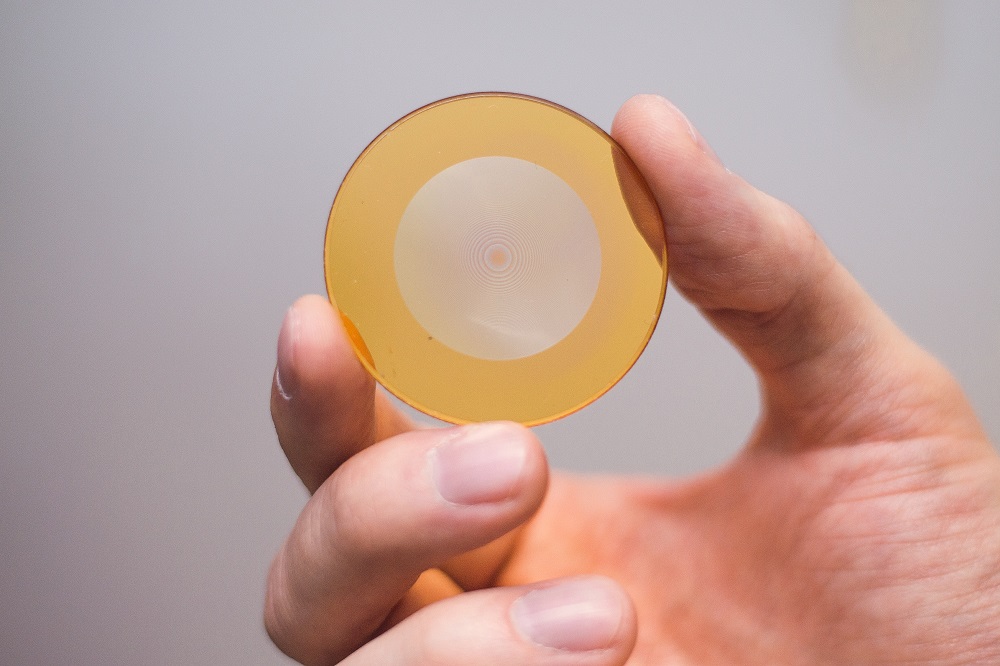
– And what is the minimum thickness of this type of zone plate?
– The zone plate is practically flat, and can have a thickness of around 1 micron, which means it can be lightweight. Let me emphasize that a zone plate only behaves like a lens at a certain wavelength. For other wavelengths, a different system of rings (Fresnel zones) is needed. This prevented the widespread use of zone plates in classical optical devices operating with white light.
– Radiation of a certain wavelength, as we know, is used in lasers...
– Yes, the creation of the laser in 1960 (winning the 1964 Nobel Prize for C. Townes, N. Basov, and A. Prokhorov), which could generate monochromatic radiation of a given wavelength, brought to the forefront the issue of creating the associated laser optics. It took some time before the new research topic was fully comprehended and began to be put in practice. My research group in Samara began to cooperate with A. Prokhorov and his colleagues, primarily with Professor I. Sisakyan, at the turn of 1970/80s.
Dealing with the issues of signal processing in information transmission channels in my candidate (1971) and doctoral (1979) dissertations, I treated the signals at the receiving end not simply as functions of time, but also of spatial coordinates, putting emphasis to their optical processing. This led to the need to design and create spatial filters for optical processors. However, in practical terms, such an approach turned out to be premature at the time. In those days, optical processors were unwieldy, not resistant to vibrations and temperature fluctuations, and could not compete in terms of accuracy with digital devices, although they provided much higher processing speeds, primarily when performing spectral transformations (so-called Fourier processors).
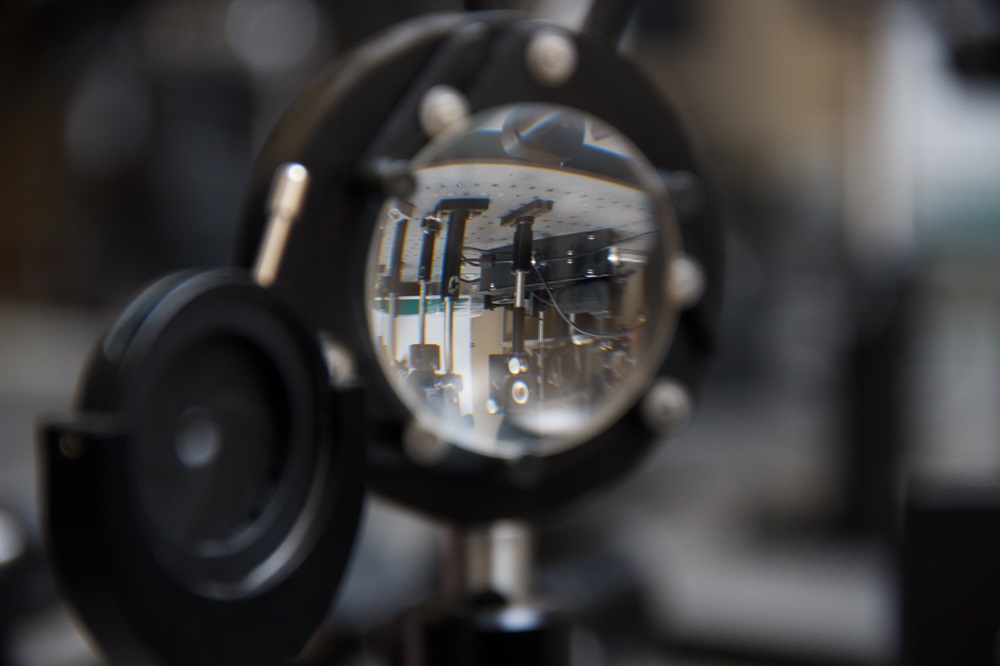
– However, despite these difficulties, your research group was able to achieve quite positive results, wasn’t it?
– An undoubted achievement of that period of time was the experience we acquired in computer design of various spatial filters and developing techniques for their fabrication based on adapted microelectronic technologies to build a diffractive microrelief. As the main achievement in this area, I consider a novel mathematical approach that we proposed and developed for the functional transformation of an optical signal – much broader than that used in classical optics at the time. Our approach is based on solving the so-called inverse problems of applied mathematics, answering the question: "What phase characteristic a spatial filter should have to be able to produce a required distribution of an optical signal at its output?" Academician A. Prokhorov highly appreciated this approach and proposed the development of a number of completely new goals for optics. The physicists breathed life into our explorations of the spatial filters. The first was the goal of focusing visible laser radiation into a longitudinal line (1980). Next (1981/82), a problem of focusing powerful infrared radiation into an arbitrary transverse intensity distribution (a ring, a cross, a square, etc.) was solved. This type of focusing was required in laser machines for materials processing (like welding, cutting, and hardening) and medical applications.
If we look at the intensity distribution in the laser beam cross-section, we will see quite a complicated spotty image with an orderly pattern. This reflects the fact that this pattern is formed by combining a certain number of so-called transverse radiation modes. These modes are mathematically described by orthogonal polynomials. In 1983, we succeeded in solving a fundamental problem of selecting transverse modes of laser radiation, which opens up fundamentally new possibilities for multiplexing optical information transmission channels and ensuring physical layer information security. In 1984, we proposed a Bessel optics method for generating vortex laser beams. This direction of research is very important for the optical information transmission in free space, scientific instrumentation, and optical micromanipulation, including microobjects in biology.
– So, you could say that this research laid the foundations for a new scientific school?
– The work I have mentioned laid the foundations for a new research direction, for which Academician E. Velikhov suggested the name "computer optics". A scientific journal with this name has been in publication since 1987, and is currently included in the 1st quartile of the Scopus database. The results of our research in the field of diffractive computer optics are reflected in the monographs "Laser beam mode selection by computer generated holograms" from Boca Raton, 1994, "Iterative methods for diffractive optical elements computation" from Taylor & Francis, London, 1997, "Methods for computer design of diffractive optical elements" from John Willey & Sons, Inc., 2002, "Vortex laser beams" from CRC Press Taylor & Francis Group, 2019, and others.
The next stage of our research was made possible by progress in the field of nanotechnologies and the emergence of microrelief recording devices with essentially subwavelength resolution (~50–100 nanometers). This dates back to the early 2000s. We started to create diffractive nanostructures with high functionality and, since it was difficult to fit the devices we synthesized into the classical subject of optics, we began to use the broader concept of "photonics" and "nanophotonics".
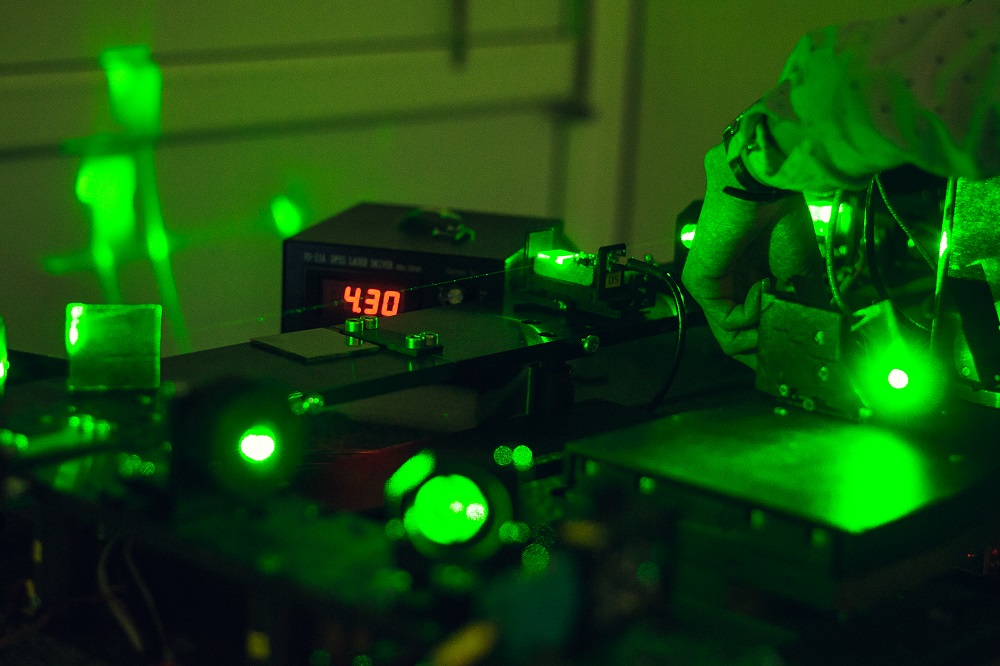
– What do these terms cover?
– Photonics looks at the interactions of optical signals with various structures, and devices based on them. Nanophotonics deals with nanostructures whose characteristic features are much smaller than the wavelengths of light they operate with. The subject of our research is diffractive nanophotonics, which studies the diffraction of light by synthesized nanostructures and devices on their basis. We are actively conducting research on the creation of resonant structures of diffractive nanophotonics for optical information processing and sensorics. It is now possible to implement them in a planar version "on a microchip". In terms of analog optical computing, the studies include the design of resonant structures for nanophotonics for optical implementation of the basic mathematical operations of differentiation and integration of optical signals, as well as more complex differential and integro-differential operators, including those for processing optical signals in "on-chip" geometry.
– Where can the results of these studies be used in practice, which fields and to resolve which problems?
– The possibility of optical realization of various mathematical operations and transformations opens up new possibilities for "superfast" solution of various problems. For example, it becomes possible to extract contours of the observed objects in real time (at the speed of light). This possibility has potential in a large number of applied problems, for example, in robotic machine vision systems.
The results of research on diffractive nanophotonics have been published in a large number of journal articles and summarized in the fundamental monograph "Diffractive Nanophotonics" from Fizmatlit, 2011, also published in English as two extended editions under the titles "Diffractive Nanophotonics" from CRC Press, 2014 and "Diffractive Optics and Nanophotonics" from Boca Raton: CRC Press, 2017.
Our research team is focused primarily on fundamental research. The leading scientists, professors L.L. Doskolovich, N.L. Kazansky, V.V. Kotlyar, V.S. Pavelyev, R.V. Skidanov, S.N. Khonina, and others boast impressively high scientometric indices. However, in the last 5–7 years, having created a number of remarkable elements of diffractive optics and nanophotonics, we have become interested in their application in instruments and systems.
Let me give you two examples. One of the results of 40 years of work by the school of diffractive optics and nanophotonics of Samara National Research University was the diffractive lens, which only weighs a few dozen grams and could replace the bulky system of lenses and mirrors in modern telescopic lenses. In May 2015, the first article to prove the possibility of using diffractive optics in imaging systems was published by Samara University’s scientists in the Proceedings of the world’s largest conference on image processing – IEEE Computer Vision and Pattern Recognition. In November 2015, a joint work on a similar topic by the University of Toronto and the King Abdullah University in Saudi Arabia was published, containing the reference to the pioneering publication of Samara University’s team. No one in the world has ever used diffractive optics to produce high-resolution color images.
When fabricating such a diffractive lens, a resist is applied to the surface of a quartz glass substrate – a photosensitive material 10 micrometers thick (for comparison, the thickness of a human hair is 40–90 micrometers). A 256-level microrelief is synthesized on the resist using a focused laser beam. In this way, an image of an object is formed, and distortion compensation is provided by computer processing the resulting images based on deep learning neural networks.
Optics based on diffractive lenses has already found applications in a wide variety of fields – space, medicine, and agriculture. The University has developed an ultralightweight optical system for remote sensing of the Earth (RSE), which will simplify and reduce the cost of creating massive groups of nanosatellites for continuous monitoring of the Earth’s surface. Miniature devices will also find their use as cameras onboard the unmanned aerial vehicles. There are plans to equip a fleet of space nanoprobes with Samara optics, which is expected to be sent to the Alpha Centauri star system as part of the Milner-Hawking project. Among the goals of this expedition is the search for potentially habitable exoplanets.
Research in the field of diffractive optical elements has allowed scientists from Samara University to create a compact hyper-spectrometer which is the basis for developing a control system for the world’s first "smart" sprinkling machine. Thanks to this system, the mobile agromeliorative complex is able to independently analyze the condition of soil while moving across a field, assessing its humidity and the content of essential mineral nutrients. Depending on the data received, the sprinkling machine autonomously adjusts the modes of irrigation and fertilization, enabling an average 25–30% increase in the crop yield.
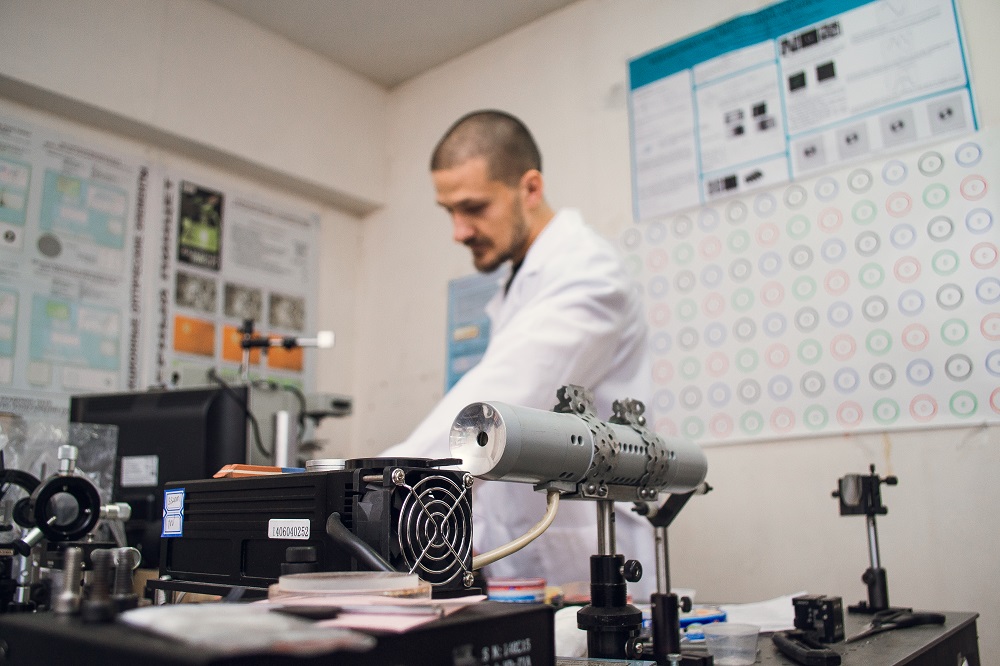
– Has Samara University, in these studies, cooperated and is it cooperating to a large extent with its foreign partner universities?
– I have to admit that at the outset of our journey into the realms of diffractive optics, our possibilities for manufacturing the elements of diffractive optics were essentially handicapped by the available technological capabilities for synthesizing diffractive microreliefs. In the period from 1985 to 2005, a positive role was played by our international cooperation with Prof. J. Turunen of Joensuu University (Finland), Prof. R. Kovarzhik and Dr. M. Duparre (Friedrich Schiller University, Germany), Dr. Piero Perlo (FIAT Research Center, Italy), and Prof. Liam O’Faolain (Cork Institute of Technology, Ireland).
These research centers boasted the most advanced equipment, prompting my colleagues to make research visits, during which a number of unique diffractive optical elements with previously unattainable characteristics were fabricated and experimentally characterized, with research results published jointly with foreign collaborators in peer-reviewed scientific journals with high impact factor.
While developing methods for designing and modeling diffractive optical elements we were also able to engage in joint research projects with foreign universities such as Leibniz University Hannover, Hannover, Germany, the IPHT research institutes and centers (Leibniz Institute of Photonic Technology, Jena, Germany), and LZH (Laser Zentrum Hannover, Hannover, Germany). Based on the research findings made, training courses were then developed, taught to students at foreign universities.
Currently, thanks to our participation in various programs of budgetary funding, the University has the state-of-the-art laboratory and experimental facilities, allowing us both to conduct research and to fabricate prototypes of diffractive optics and nanophotonic devices. These days, the international cooperation of the University shows no signs of decline; on the contrary, it is gaining momentum. Dr. D. Nesterenko is working in close cooperation in the field of photonic sensorics with professor Hayashi (Kobe University, Japan). Professor S. Khonina is actively cooperating with Prof. Korotkova (University of Miami, USA) in the field of the generation and study of the propagation of vortex beams. Cooperation of Samara University with its partner universities gives the greatest effect when performing the most complex experiments in diffractive nanophotonics. For example, the results of an article published in June 2020 in the Optics Letters journal with the participation of Dr. A. Porfiryev and Professor S. Khonina were made possible due to extensive cooperation with both domestic and foreign universities (Swinburne University of Technology, Hawthorn, Australia; Tokyo Institute of Technology, Japan; Ruhr-Universität Bochum, Germany; University of Applied Sciences Münster, Germany).
The research direction associated with the development of nanophotonic structures for optical information processing and optical computing systems is on the research agenda of the world’s leading universities (Massachusetts Institute of Technology, Stanford University) and research centers (IBM Research). This provides potential opportunities for scientific interactions with leading universities and research centers.
– Does research in this field receive support from the government and business?
– I have already said that the work in the field of diffractive optics and nanophotonics is carried out in close cooperation between the Research and Education Center and the Image Processing Systems Institute of the Russian Academy of Sciences from the very beginning. The Institute is funded by the Ministry of Science and Higher Education of the Russian Federation. We also receive research grants from the Russian Foundation for Basic Research and the Russian Science Foundation, and the Innovations Development Assistance Fund. Our research is also financially supported by the government of the Samara Region. The Competitiveness Enhancement Program of Samara National Research University provides funds for purchasing equipment and awarding scholarships to high-performing researchers.
The development of methods for designing and modeling diffractive optical elements has also earned the Institute a number of commercial contracts with companies and organizations for the development of the software for diffractive optics and nanophotonics. These include the FIAT Research Center (CRF FIAT, Italy), Hitachi Via Mechanics (USA), Oy Modines Ltd. (Finland), Institute of Optics, Berlin University (Germany), and others. Joint research work is being carried out in the field of diffractive optics for the terahertz range with the Russian company Tydex LLC (St. Petersburg). Joint research has been carried out in the field of infrared diamond diffractive optics with the General Physics Institute of the Russian Academy of Sciences (Moscow). The created elements of diffractive optics in the terahertz range were experimentally characterized using a unique scientific mega-installation – a free electron laser (NOVOFEL) at the Institute of Nuclear Physics of the Siberian Branch of the Russian Academy of Sciences (Novosibirsk).
– What plans are there for the future? Will this research be continued?
– Samara National Research University is now working out a development strategy until 2030. Scientific and educational activities in the areas I mentioned in this interview will have a significant place in this strategy. At present, the University is planning to participate in two serious competitions of the Ministry of Science and Higher Education of the Russian Federation. Both competitions were announced by Minister V.N. Falkov. This is the "Academic Excellence" competition aimed at integrating universities with the RAS institutes, as well as a competition of world-class scientific and educational centers. In the Samara region, by order of the governor D.I. Azarov, a Research and Education Center "Engineering of the Future" has been established, and our University is a part of it. This REC will participate in the federal competition for the status of a world-class scientific and educational center. We will continue to carry out fundamental research and create devices by integrating diffractive optics and nanophotonics with artificial intelligence.
– What results are you hoping for?
– We plan to achieve the following main results:
- create resonant nanophotonic structures for the implementation of optical sensors in the "on a microchip" configuration;
- propose new types of light beams that carry orbital angular momentum (OAM), including fractional OAM, for free-space communication systems with physical layer security of the transmitted information;
- develop end-to-end optical-informational technologies for the intelligent processing of ERS data to address problems related to precise agriculture, monitoring and improving the quality of the living environment;
- fabricate experimental prototypes of wide-aperture imaging lenses based on diffractive lenses for the use in the Earth remote sensing (ERS) systems.
A promising direction in the development of scientific research is the use of diffractive optics methods for controlling long-wave coherent radiation in high-power installations of the terahertz and microwave ranges for solving problems of megascience and high technologies.
We will also continue the work on all-optical analog computing. Mobile devices based on this principle are very promising and have great advantages over electronic counterparts in terms of operation speed and noise immunity.
We will continue to work on adapting our devices for operation onboard the "CubeSats". We already have good preliminary results in this direction. The competences of the scientists of Samara National Research University in the field of diffractive optics and nanophotonics are attracting considerable interest from industrial enterprises and government agencies in areas such as monitoring the living environment, smart farming, robot vision, etc.